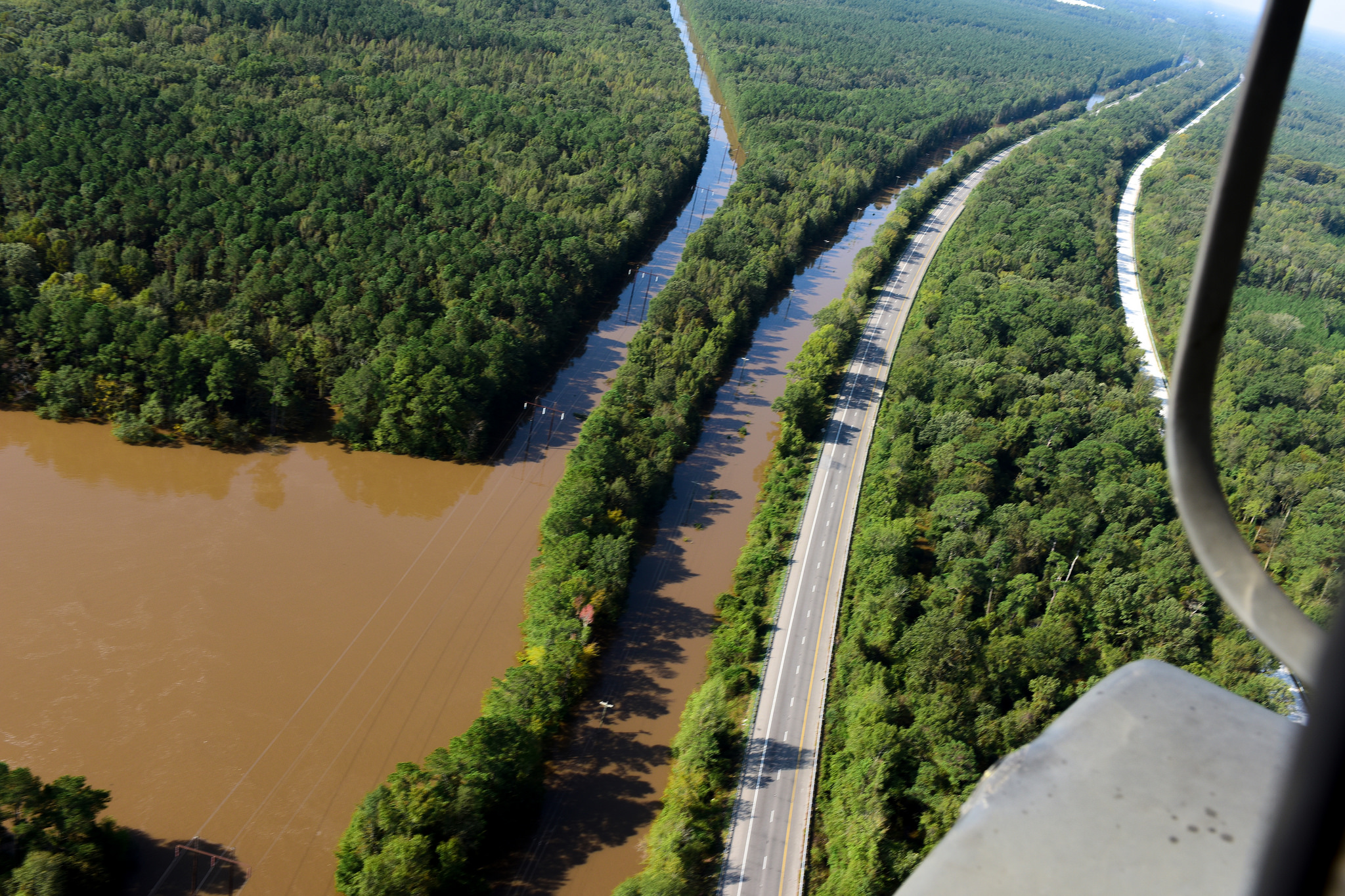
Flooding caused by Hurricane Florence in South Carolina in September. Engineering programs need to ensure that students have a firm grasp of technological, social, and sustainable systems theory and principles, big data and artificial intelligence, and cybersecurity and cyberwar operations SC Air National Guard / Flickr
Brad Allenby is President’s Professor of Sustainable Engineering and Lincoln Professor of Engineering and Ethics at Arizona State University. Mikhail Chester is an associate professor of civil, environmental, and sustainable engineering and director of the Metis Center for Infrastructure and Sustainable Engineering at Arizona State University.
Republished with permission from `Issues in Science and Technology`, Allenby and Chester, “Reconceptualizing Infrastructure in the Anthropocene,” Spring 2018, p. 58-63, by the University of Texas at Dallas, Richardson, TX.
In a world where natural systems are for many purposes becoming simply another element of regional and global infrastructure systems, society in general and engineers in particular are challenged to rapidly develop tools, methods, and intellectual frameworks that enable reasoned responses. The reductionism inherent in pretending the challenge is just climate change or new transportation systems or lower-carbon energy production and distribution systems or water quality and quantity, although appropriate in certain cases, can no longer be defended as an acceptable disciplinary or institutional approach to infrastructure design, operation, and management. Rather, engineers, engineering educators, and institutions that support and manage infrastructure need to create solutions in the real world, and to embrace the implications of the Anthropocene. Bluntly, the world is a design space, and absent catastrophic collapse, there is no going back.
The reality of the terraformed Earth, whatever one wants to call it, requires some significant changes in the way technology and infrastructure are designed, implemented, understood, and managed. Although the obvious target disciplines are in engineering, such a focus would be inadequate. Increasingly, private firms develop and manage emerging technologies that shape the integrated human/built/natural infrastructures that are the defining characteristics of the Anthropocene, so business education writ broadly must be part of the shift. Moreover, as systems that were previously considered external to human design, such as biology and material cycles, increasingly become design products, many aspects of science segue into engineering fields, requiring different mental models and tools. This implies that many fields of science also require some grounding in technology, design, and engineering. Against this background, and recognizing that any suggestions for reform at today’s early stage will necessarily be partial and somewhat arbitrary, we can nevertheless make some recommendations for change. For simplicity, we will group these suggestions into three categories: short term, medium term, and long term.
The growing impact of human activities on all the Earth’s systems requires a concomitant change in the way we design and manage the built environment.
A fundamental shift is afoot in the relationship between human and natural systems. It requires a new understanding of what we mean by infrastructure, and thus dramatic changes in the ways we educate the people who will build and manage that infrastructure. Similar shifts have occurred in the past, as when humanity transitioned from building based on empirical methods developed from trial-and-error experience, which was sufficient to construct the pyramids and European cathedrals, to the science-based formal engineering design methods and processes necessary for the electric grid and jet aircraft. But just as the empirical methods were inadequate to meet the challenges of nineteenth- and twentieth-century developments, today’s methods are inadequate to meet the needs of the twenty-first century. Now that we have entered the Anthropocene period in which human activities affect natural systems such as climate, engineers face far more complex design challenges.
The educational shift is not unlike the institutional shift. Engineering education in particular combines quantitatively complicated but structured models and learning with creativity, design, operation, and problem-solving capability. The former is rapidly becoming mechanized, and the latter is increasingly done in a rapidly changing social, economic, and technological environment. Because of this, it will not be enough to simply reform engineering education in the short term: rather, engineering education must become a constantly evolving process and curriculum that changes at the same pace as its context. Engineers, who today often resemble expert systems—highly competent in one specific area such as chemical or civil engineering, but with knowledge that rapidly scales off at the edge of that domain because they don’t have time to gain much education outside their particular discipline—must instead become constantly learning quantitative problem solvers, able to integrate across many different domains, as well as the still yawning gap between the science and technology fields and the social sciences and humanities.
These changes sound challenging, and indeed they are. But the Anthropocene is not a choice that we can reject; it is a reality that we have often unintentionally been creating since the Neolithic. We are now at the point where it must be embraced in all its complexity if we intend to respond rationally, ethically, and responsibly to its challenges.
These emergent properties would not exist but for humans; the design process involved, however, is not the explicitly rational and quantitative method that we are used to. Rather, a planet characterized by rapidly increasing integration of human culture, built environments, and natural systems producing novel, highly complex, rapidly changing emergent behaviors challenges all our existing ideas about design, operation, and management of infrastructure. Indeed, it challenges the very idea of infrastructure as limited to local, highly engineered systems. For in such a world, “natural” cycles and systems transmute from exogenous conditions into infrastructure components, a process implicitly recognized by, for example, the substantial literature on ecosystem services and earth systems engineering and management.
Consider urban water supply. Replacing a water pipe in New York City, albeit expensive and complex, is well within current professional and institutional capabilities. On the other hand, to maintain that water supply means designing, through engineering and land use regulation, a continuously monitored network of 19 reservoirs in a roughly 2,000-square-mile watershed. Similarly, most people know that Arizona’s population is concentrated in several large urban areas in semiarid desert regions of the state. Contrary to popular belief, however, Arizona’s water supply is robust. It is very diverse, with 17% from in-state rivers and associated reservoirs, 40% from groundwater, 3% reclaimed, and 40% imported from the Colorado River, which channels water from seven states. Flexibility and resilience in operations is enhanced because imported water is largely banked in underground reservoirs, while wastewater is highly managed and either returned to the ground where it can be accessed later or sent to power generation facilities. Similarly, water in California is tightly designed. Mountain snow releases water as it melts, and that runoff is heavily managed for multiple uses as it moves toward the ocean. As in many other regions, natural flows from precipitation are now highly managed and there is increasing recognition that historical flow patterns are becoming less and less relevant for predicting future flows. In short, watersheds are now so highly managed that the environment has in effect become the water infrastructure.
The durability of the pyramids and cathedrals is evidence that the inherited wisdom of experience can be adequate for many tasks, but we can also see the disadvantages of working without the formal design tools based on advanced mathematics and engineering science. The choir vault and several buttresses of the Beauvais Cathedral in France collapsed in 1284, only 12 years after its partial completion. Retrospective analysis suggests that the problem was resonance under wind load, the type of stress that could be anticipated only with the formal design methods that were yet to be developed. And when the first iron bridge was built at Coalbrookdale, England, in 1781, the engineers used the same design they would have used for a masonry bridge. Within a hundred years, however, structures such as James Eads’s 1867 bridge over the Mississippi River at St. Louis and Gustave Eiffel’s 1884 Garabit Viaduct were being formally designed using scientific methods and quantitative design processes that made it possible to predict the performance of the new materials. Personal experience and historical heuristics were replaced by scientific data and quantitative models. Opportunities for infrastructure evolution that would have been impossible without scientific understanding suddenly became available, leading to more efficient, effective, and safer infrastructure, and eventually to entirely new forms of infrastructure such as air travel and electrification.
The engineering tools, methods, and practices necessary for a practicing professional; the engineering education necessary to prepare such a professional; and the way engineering is performed institutionally have all changed fundamentally throughout history as the context within which humans live and prosper has changed. We are now in a period of rapid, unpredictable, and fundamental change that is literally planetary in scope. To date, neither our ideas regarding infrastructure, nor our educational and institutional systems for conducting engineering, have reflected this striking evolution in context. We are well past the point where adaptation is pragmatically, and ethically, required.
It is important to remember that this doesn’t mean that all current practice and educational methods are suddenly obsolete. Rather, it means that they are increasingly inadequate, especially when applied to projects that involve complex social, economic, and cultural domains. Designing a water treatment plant will remain well within the capability of today’s field of environmental engineering; designing the nitrogen and phosphorus cycles and flows in the Mississippi River basin to reduce the life-depleted “dead zone” in the Gulf of Mexico cannot be done so simply.
That we now live on a terraformed planet is not a new idea. The term “Anthropocene” was popularized in an article in 2000 by Paul Crutzen and Eugene Stoermer, but as early as 1873 the Italian priest, geologist, and paleontologist Antonio Stoppani used the term “anthropozoic era,” and others have used similar language throughout the twentieth century. The force driving the birth of a new era is obvious. A planet that supported roughly 450 million humans in 1500 now supports over 7.5 billion, and because of industrial progress and economic growth, each of those humans has a vastly more consequential impact.
In a blink of geologic time, then, humans left a planet where they were but one species among many and built a world increasingly shaped by the activities of, and for the purposes of, a single species—themselves. It is not that the planet has been deliberately designed by humans, but many human-built systems from transportation to communications have resulted in global changes that were not consciously designed by anyone. Moreover, that human activity is clearly a significant contributor to current dynamics of such natural phenomenon as climate change, biodiversity, nitrogen and phosphorous cycles, microbial evolution, and regional ecosystem parks such as the Everglades does not imply deliberate design in such cases either. Rather, the world we find ourselves in is one where deliberate human activity, itself a complex network of functions, interacts with a highly complex planetary substrate to create unpredicted, often challenging, emergent behaviors, of which climate change is but one example.
Short term
In all relevant disciplines, including at least all engineering and business programs, at least three areas of learning should be mandatory for all students, undergraduate and graduate, and professional accreditation institutions such as the Accreditation Board for Engineering and Technology (ABET), which approves engineering programs, should set an explicit timetable for achieving this. Whether these areas of expertise are introduced as modules in existing curricula or as independent courses is not as important as ensuring that some level of substantive knowledge is acquired by all students. In order to reinforce this, professional exams, such as the Fundamentals of Engineering, and Principles and Practice of Engineering exams given to professional engineers, should be expanded to include these subject areas. These broad areas of competence are:
- Technological, social, and sustainable systems theory and principles, including concepts such as wicked complexity and satisficing versus optimizing system performance. This category of knowledge includes understanding the difference between simple and complex system behavior, and designing and managing integrated human/built/natural systems.
- Big data/analytics/artificial intelligence (AI) functions and systems. Any business or engineering student who graduates without knowing something about these fields is verging on incompetent.
- Cybersecurity and cyberwar operations from a defensive and offensive perspective. Today, business managers and engineers are trained to rely on, and design and deploy, “smart” systems at all scales; indeed, in many cases this is a performance and business imperative. On the other hand, virtually none of these professionals are taught anything about cybersecurity or cyberwar, a gaping competency chasm when both Russian and Chinese strategic military doctrines have shifted toward deployment of unrestricted warfare/hybrid warfare/cyber warfare initiatives. Inviting an adversary to subvert all your critical infrastructure and systems, which the US educational system now does, is simply stupid, especially since these adversaries are very engaged, and quite competent, in such activities.
Long term
The longer-term situation is more inchoate and more complex. Three fundamental shifts, one conceptual, one systemic, and one educational, are required, but none of them can be planned with any specificity. Accordingly, an overriding requirement is to learn how to implement continuous dialog and learning with the many systems within which we are embedded so that we can adjust our mental models, institutions, and educational practices in response to real time changes in system state. Policy and practice become processes of adaptive evolution rather than static responses to a stable world, and technocrats, managers, and engineers must learn to focus on ethical and responsible processes, and less on content, which will be continually and unpredictably changing.
The conceptual shift sounds simple. The fundamental implication of the Anthropocene is that everything from the planetary to the human body itself become design spaces: the world has become infrastructure. Especially given the complexity of the systems involved, this doesn’t mean design in the sense of a completely understood controlled system such as a toaster. The world of simple, optimizable design is gone. Rather, it means that in a world of radical human life extension, integrated AI/human cognition, ecosystems as infrastructure, and re-wilding using previously extinct species in accord with wilderness chic design aesthetics, everything is subject to human intervention and intentionality. The Everglades is a design choice and exists today in its current state because we choose to have it exist in that state, even if it obviously has biological and ecological complexity beyond our current understanding. The simple truth of a terraformed planet is difficult for many people to accept, for reasons ranging from fear of the responsibility to ideological commitment to archaic visions of the sanctity of nature, but it is a necessary basis for technocratic and policy professionals if they wish to act ethically and responsibly under today’s extremely challenging conditions.
Systemically, institutions need to evolve to mirror the complexity and interdisciplinarity of the networks with which they are interacting. Importantly, this means that institutions must become multi-ideological if they want to manage regional and planetary infrastructure. If they do not, they will be ineffectual. Thus, for example, the United Nations Framework Convention on Climate Change, signed in 1992, prioritized environmental ideology over other values, with the result that implementation has been sporadic, contentious, and ultimately unsuccessful. The shift involved here is also conceptual, and deceptively simple: institutions driven by activist stakeholder and dominant ideological demands must instead begin to frame themselves as problem-solvers, with the desired goal being not the advance of a particular agenda, but the creation of a politically and culturally stable solution to a particular challenge. Moreover, the institutions that typically govern infrastructure are mechanistic, characterized by hierarchical structure, centralized authority, large numbers of difficult to change rules and procedures, precise division of labor, narrow spans on control, and formal means of coordination. In contrast, industrial organizations in sectors exposed to rapid and unpredictable change, such as Silicon Valley technology firms, are far more organic, with the emphasis on moving fast rather than the lumbering pace imposed by formal structure. Whether current infrastructure institutions are capable of transitioning their management structures is an open question, given their legalistic and bureaucratic incentive structures; if they cannot, they must be replaced.
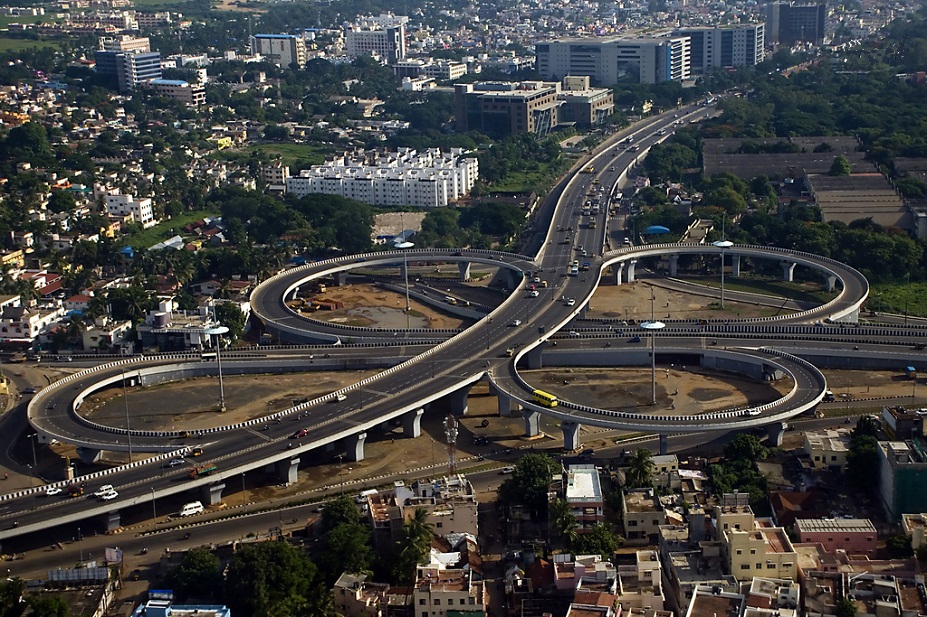
Kathipara Junction, Chennai, is Asia's largest cloverleaf. The world is a design space, and increasingly, private firms develop and manage emerging technologies that shape it. Besides engineering, business education must also be part of the shift. Pratik Gupte / Wikimedia Commons
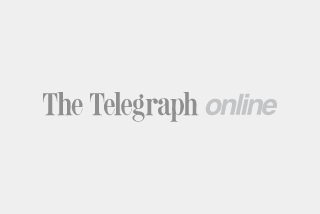
The first iron bridge, built at Coalbrookdale, England, in 1781. Its engineers used the same design they would have used for a masonry bridge. Within a hundred years, however, structures were being designed using scientific methods and quantitative design processes that made it possible to predict the performance of the new materials James Humphreys / Wikipedia
These changes in curricula are a one-time improvement and by themselves will quickly become inadequate. At least as regards engineering, two further steps should immediately be taken. It is, for example, sadly the case today that too many engineering students are still being graduated with skill sets that prime them for replacement by expert systems. Thus, the first step should be to review the entire engineering curriculum for each discipline, identifying those elements of existing courses that software, AI systems, and changes in technology have made obsolete. A thorough analysis of the content of existing courses, coupled to modernization of ABET criteria, will help create educational programs that prepare students for the future, not the 1950s.
This should be combined with implementation of a change that engineering schools and those who hire engineers have been talking about for many years but have yet to implement: making engineering a graduate-level professional degree. Uniquely among professions, engineering remains, at least in theory, an undergraduate professional degree: neither law nor medicine nor any other major profession does so. This anachronism today works only by impoverishing the scope and scale of the education provided to undergraduate engineering students, who are then thrust into a world that demands ever more operational sophistication from them—something they cannot provide because there’s no room for anything but engineering-related courses in their undergraduate programs.
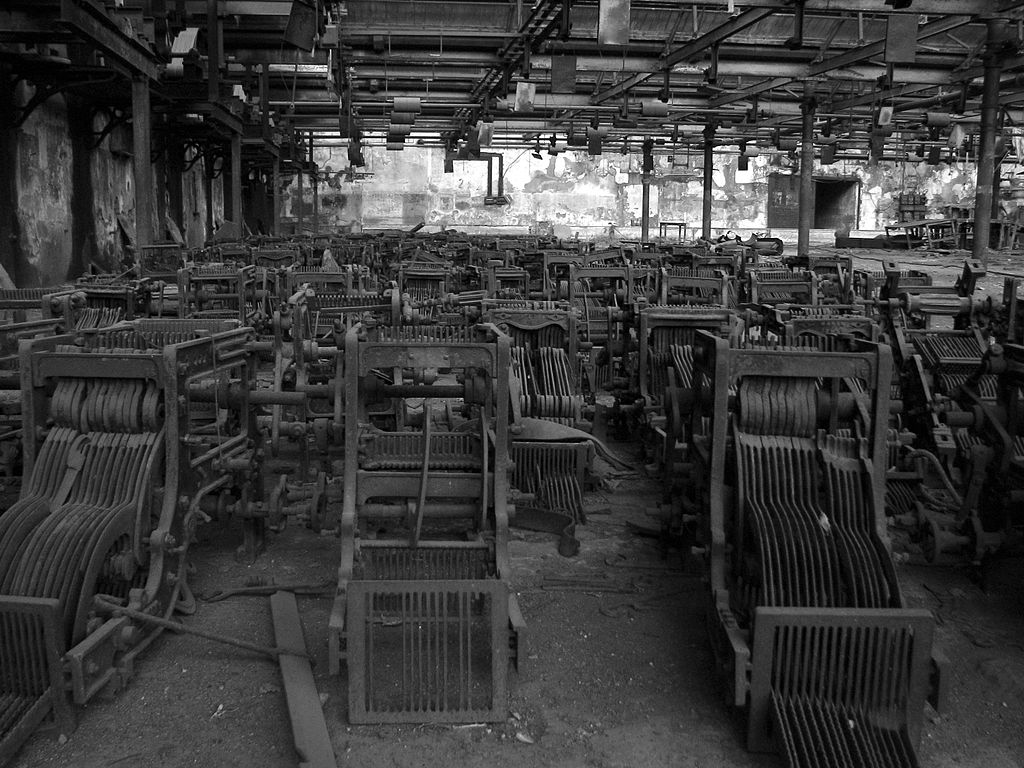
A 2008 file photo of an abandoned mill in Mumbai. We cannot stop building infrastructure, but given that many forms of the built environment outlast the context in which they were built, we can try to design infrastructure to be more adaptable and agile. Kunal Ghevaria / Wikimedia Commons
Medium term
The Anthropocene is characterized by unpredictable change with far shorter time cycles than those common to most infrastructure systems. An important emphasis, therefore, must be on developing frameworks, tools, and methods that enable more agile, adaptable, and resilient infrastructure design and operation even in the face of fundamental uncertainty about virtually every important challenge infrastructure systems will face.
Although specifics are difficult and remain to be worked out, there are a number of ideas and models across existing engineering systems that can be generalized toward the goal of agile, adaptive, and resilient infrastructure. For example, the entire domain of consumer computational technology is characterized by many different technologies that must converge in working systems that are robust and simple enough in operation for average consumers to understand. One of the basic mechanisms for this process is “roadmapping,” where modularity of technology—printers are different from data storage devices are different from input devices—is combined with robust module interfaces to enable both module and overall product evolution that is at once unpredictable, functional, and economic. Another example from consumer electronics is the substitution of software for hardware functionality whenever possible. Assuming appropriate hardware design, software can easily be upgraded, whereas hardware is far more difficult to change out (imagine if every improvement in software security or function required a new motherboard!). And programming offers a third model: the core of C++, for example, has remained fairly stable for years, but software evolution based on that core has been explosive, unpredictable, and very rapid. This suggests a “hub-and-spoke” framework: those elements of an infrastructure system that are not likely to change rapidly can be built with longer time frames and be more robust, whereas the spokes and edge elements, connected with stable interfaces, can evolve unpredictably and much more rapidly. Other characteristics might include planned obsolescence, resilience thinking that includes safe-to-fail and extensible design that can be easily updated, and increased compatibility and connectivity of hardware. These and similar models should be applied to infrastructure design generally.
As these examples suggest, the advent of the Anthropocene requires the development of new institutions and frameworks that are beyond today’s traditional disciplinary structures and reductionist approaches. Continued stability of the information-dense, highly integrated, rapidly evolving human, natural, and built systems that characterize the anthropogenic Earth requires development of new abilities to rationally analyze, design, engineer and construct, maintain and manage, and reconstruct and evolve such systems at local, regional, and even planetary scales. It is not that current institutions and disciplinary boundaries are completely obsolete; rather, it is that increasing complexity and rates of system evolution require a new and more integrative level of sophistication in infrastructure conceptualization, design, and management. This can draw on a number of relatively new fields of study, including sustainable engineering, industrial ecology and associated methodologies such as life-cycle assessment and materials flow analysis, systems engineering, adaptive management, and parts of the urban planning and sociology of technology literatures.
The boundaries reflected in current engineering institutions, disciplines, and educational practices and structures are still appropriate for many problems. But today, at the dawn of the Anthropocene, they increasingly fail at the level of the complex, integrated systems and behaviors that characterize the anthropogenic Earth. No disciplinary field in either the physical or social sciences addresses these emergent behaviors, and very few even provide an adequate intellectual basis for perceiving, much less parsing, such complex adaptive systems. Engineering cannot continue to rest on traditional curricula and strengths, which are increasingly inadequate given today’s social, economic, environmental, and technological demands. A road built into a rain forest to support petroleum extraction operations cannot be well designed unless it is understood that such infrastructure becomes a corridor of development and environmental degradation. Similarly, a new airport planned in Peru in the Inca Sacred Valley cannot be adequately designed unless its role in dramatically increasing tourism and development in a fragile ecosystem and historic cultural region is understood, and the numerous effects evaluated. Planning for urban transportation infrastructure is inadequate unless it includes consideration of the implications of such varied developments as hybrid, electric, and autonomous vehicle technologies, potential climate change effects on mobility, aging populations, and terrorism reduction and mitigation. We cannot stop building infrastructure, but especially as many forms of the built environment outlast the context within which they were built—think of coal-fired power plants being built today that will be operating for decades—we can try to design infrastructure to be more resilient to change even when we cannot predict the particulars of such change, to be more adaptable and agile as those changes occur, and to be more aware of obvious effects of our designs that may have heretofore been ignored.
Because of their importance to society, infrastructure systems tend to be designed to be robust and long-lasting. But especially as “natural” systems increasingly become a part of large infrastructure design and planning, it may be necessary to identify infrastructure where context may be changing rapidly, and “down-design” it: that is, design it to be less robust, more temporary, and capable of being replaced inexpensively and much more quickly. This could be combined with a major hubs/minor hubs/links design, where robust major hubs last for long times, minor hubs are down-designed, and structural links are robust in the face of unpredictable challenge.